This application note illustrates the effect of flow generated by a peristaltic pump vs a pressure-based system applied to a microfluidic system’s cell culture.
Introduction
The development of microfluidic research applied to cell culture has revealed the need to generate more accurate propulsion systems with high stability and pulseless flow. In this way, pressure pumps emerge instead of peristaltic pumps that have been widely used but create alterations in the flow rate. Precise microfluidic pressure systems allow controlling both pressure and flow rate to avoid these intense pulses. Although one of the main disadvantages of the pressure pumps was the limitation of the liquid volumes they could dispense; currently, the recircularization systems allow distribution volumes and solve this limitation. Thus, pressure pump systems offer more technically advanced solutions to microfluidic applications.
Material and Methods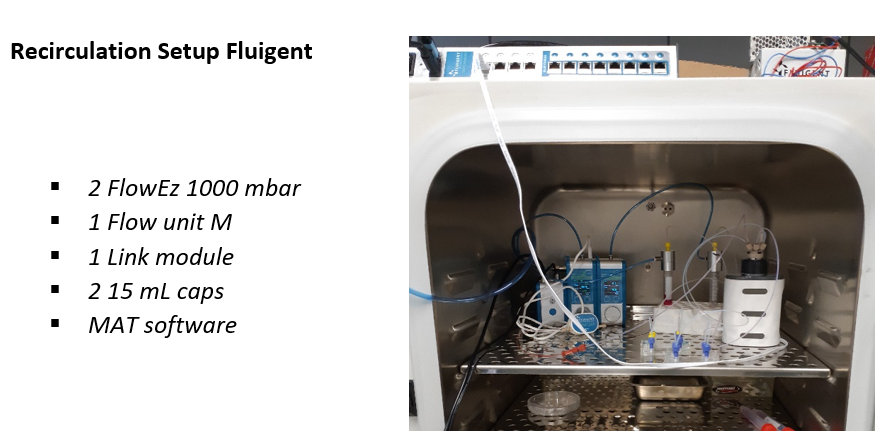
The pressure pump system consists of the following elements: a 1000 mbar FlowEZ pressure controllers, a FlowUnit M to monitor flow rate, L- switch module which allows media recircularization combined with a LINK module to enable a connection with a PC. The programs used to control this system’s different elements were: All-in-one, Microfluidics automation tool, and ESS control.
In both systems, the flow rate was monitored with a sensor to evaluate possible fluctuations.
Brightfield and fluorescence microscopy were used to visualize cells before and after flow (Nikon Ti-E microscope). Nuclei were stained with Hoestch, a blue fluorescent dye. Image analysis was performed using FIJI software.
Protocol Steps
To compare both flow systems, Be-flow microfluidic devices (Beonchip) were seeded with endothelial cells (HBEC-5i: ATCC® CRL-3245™). HBEC-5i cells grow using DMEM-F12 culture media supplemented with 10% FBS, 40 µg/mL endothelial growth supplement, and 1% antibiotics.
Steps:
- Coat the Be-Flow channels with a solution of 0,1 mg/mL collagen type 1 at 37ºC
- Seed cells in a suspension until a monolayer is formed.
- Connect the Be-Flow to the µfluidic system following the instructions of this link.
- Set the flow rate at 50 µL/min and leave it working in this condition for 24h inside an incubator at 37◦, 5% of CO2.
Results
Our experimental conditions’ flow-time curves show that the peristaltic pump presents less stability and intense fluctuance, such as a lack of responsiveness (Figure 1). That’s critical because these strong and unbalanced pulses of over 30% of the flowrate set can induce cell detachment. On the other hand, a pressure pump is the best option for flow-rate control solutions, showing minimum flow rate variations bellow 1 % of the set value.
Figure 1: Flow-time curve. Curves show the flow variations along time with a fixed 50 µL/min flow rate.
Microscopy images show that most endothelial cells lose their adhesion after 24 under peristaltic flow conditions, and cellular density has decreased significantly. The remaining cells show morphological changes related to low adhesion or cell deterioration (Figures 2 and 3). However, with the pressure pump, cells present a similar morphology before and after the flow assay. Moreover, there are no significant differences concerning cellular density (Figures 2 and 3).
Figure 2: Contrast and fluorescence microscopy images of HBEC-5i cells seeded in Be-Flow microfluidic devices before flow. Nuclei are stained in blue with Hoestch dye. Upper row: Pressure pump at 0 hours. Lower row: Peristaltic pump at 0 hours. Scale bar is 100 μm.
Figure 3: Contrast and fluorescence microscopy images of HBEC-5i cells seeded in Be-Flow microfluidic devices after 24h of flow at 50 µL/min. Nuclei are stained in blue with Hoestch dye. Upper row: Pressure pump after 24 hours. Lower row: Peristaltic pump after 24 h. Scale bar is 100 μm.
The results show that pressure pumps are less aggressive for cells in the same practical conditions and let them stand adhered for more time.
Conclusion
Pressure-based microfluidic flow control systems represent a much more effective alternative to classical peristaltic pumps, showing better results in controlling the flow and avoiding intense pulses. This precise and continuous flow keeps the cells adhered and, therefore, preserves the system to carry out long-term tests.