Summary
Gravity-driven flow is paramount during the first stages of culture in organ-on-chip platforms. This study demonstrates oxygen consumption in a cellular monolayer within a microfluidic device and highlights the significance of gravitational-flow in maintaining optimal oxygen levels during the early stages of cell culture with COP devices. These findings emphasize that gravity-driven flow is crucial before integrating a flow control system.
Introduction
Cyclic olefin polymer (COP) is a material with very low gas permeability that Beonchip uses to manufacture its devices. It is a very interesting medical-grade plastic, that offers very low gas permeability, resistance to solvents, acids and bases, heat resistance, excellent optical properties, and no issues with nonspecific adsorption [1].
In addition to these advantages, COP allows control over gas concentration within the devices. This provides an advantage in developing certain in vitro models where gas concentrations, such as oxygen, play a key role in the development of the microenvironment and physiological phenomena like tumor microenvironments, ischemic processes, or anaerobic conditions [2].
Oxygen is essential for life, driving metabolic processes that support homeostasis and cellular function. However, it also poses risks of cellular damage, requiring a careful balance between its use and antioxidant defenses. Thus, alterations in oxygen concentration can lead to dysfunctional processes, resulting in pathological oxygen reduction or deprivation known as hypoxia, recognized as a hallmark of many diseases (e.g., ischemia, tumor microenvironments).
Depending on the type of tissue and organ, oxygen pressure varies, exposing different cell types to unique gas conditions [3]. Replicating these conditions is important for achieving a more realistic cellular response.
This study characterizes the oxygen consumption of a cellular monolayer in a microfluidic device and examines how gravity.driven flow flow helps maintain optimal oxygen concentrations during the initial phases of cell culture using thermoplastic Organ-on-chip devices.
Methodology
Be-Flow
The design of the Be-flow consists of 2 independent channels with screw-like inlet and outlet wells that allow joining with connectors and tubing compatible with any fluidic system.
Therefore, It is possible to apply an independent flow rate in both channels using our patented inlet/outlets and medium reservoirs that avoid the entrance of bubbles into the channel.
Evaporation reservoirs are situated next to the medium reservoirs to be filled with PBS/water during the incubation before closing the system to a flow control system.
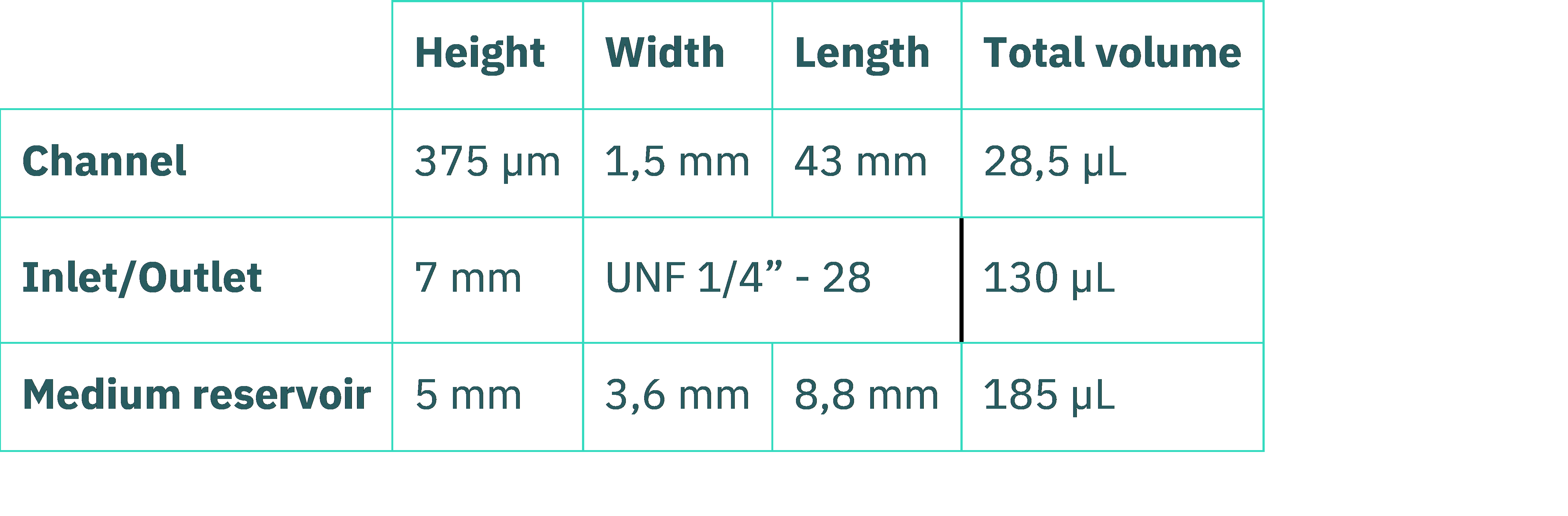
Table 1: Be-Flow feature dimensions
Rocker (Gravity-driven flow)
As microfluidic main purpose is to reduce sample and size, the medium volume of the channels is limited. To promote optimal cell growth, it is essential to refresh the supply of nutrients and oxygen. Therefore, to replenish the cell culture medium (after cell attachment but before connecting any perfusion system) the rocker (Figure 1) constitutes a practical tool (video). The tilting displaces the medium back and forth from one medium reservoir to another through the channel. This is a gentle flow that may vary according to the channel dimensions, speed motion and rocking angle.
The Rocker allows the use of multiple devices simultaneously.
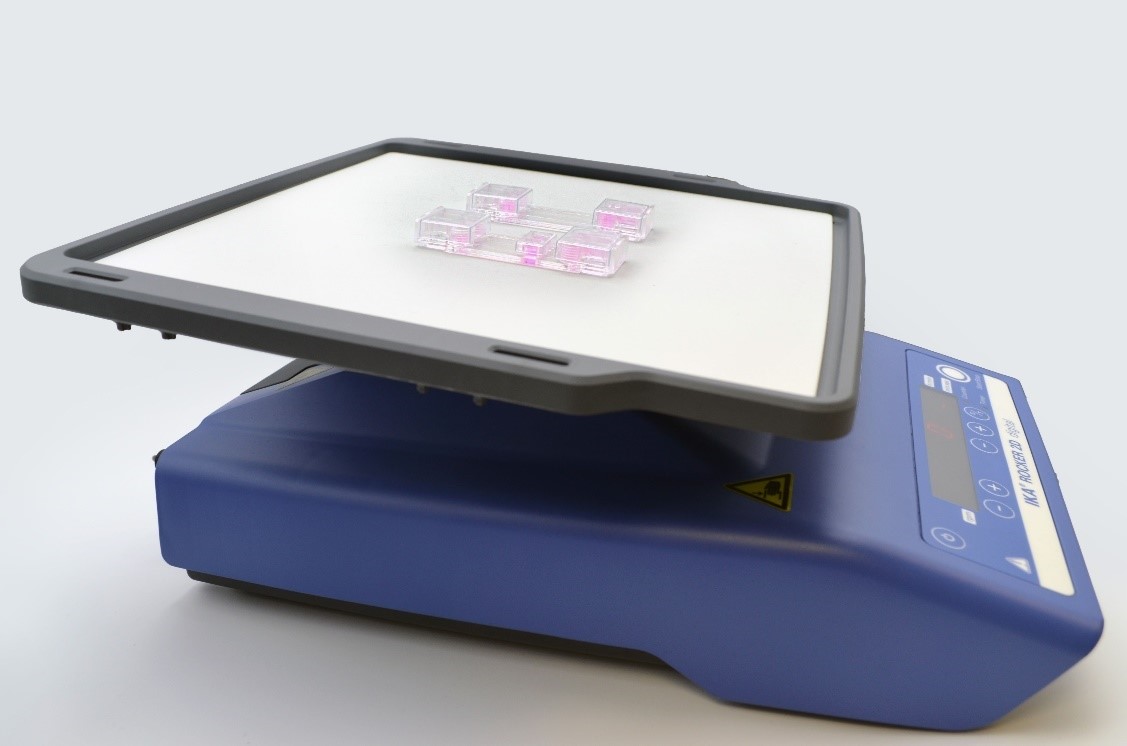
Figure 1. IKA Rocker 2D digital with Beonchip devices.
Cell culture
Before cell seeding, pre-warm the device in the incubator overnight to minimize air bubble formation.
- Fill the channel with 100μl of collagen at 0.1 mg/ml (in PBS 1X) and incubate at 37°C for 30 minutes. Take a look in this video tutorial how to make a coating in Be-Flow.
- Remove the coating completely emptying the channel. Seed 106 Caco-2 cells resuspended in 35 μl of culture media.
- Incubate at 37°C, 5% CO2 in static conditions until cell attachment (2-4h). Our chip lids are very useful during this step.
- Add 300μl of culture medium into the medium reservoirs.
- Add H2O/PBS to the evaporation reservoirs, cover and keep the device in the incubator under gravity-driven flow using a rocker. For the control device keep the roicker conditions static.
During the 15h of cell cuture we took oxygen measurements using Presens sensors every 5 minutes.
Results and conclusions
Oxygen concentration measurements in static vs gravity-driven flow condidions
This study analyzes the effect of gravity-driven flow on the initial stages of cell culture and the oxygen fluctuations within a channel cultured with a monolayer of epithelial cells (Caco-2). Figure 2, shows that oxygen concentration (blue line) decreases to 2% O2 after four hours due to cellular consumption and metabolism under static conditions. In static conditions, there is no renewal of the culture medium. This makes the medium within the channel their only source of oxygen and nutrients. Consequently, due to the gas impermeability of the chip materials, the oxygen concentration decreases as a result of cellular activity.
In addition, we analyzed the oxygen profile within a channel with a monolayer of epithelial cells under constant medium refreshment. This was done by comparing the previous static culture condition (blue line) with a dynamic culture condition (orange line), which involves renewal of the culture medium in the channel (the region where the cells are located) using a rocking platform. In this way, the culture medium in the reservoirs located at each end of the channel (which exchanges gases with the incubator atmosphere) moves through the channel, supplying atmospheric oxygen levels to the cell culture. Figure 2 shows how oxygen levels are thus maintained between 16% and 12% throughout 15h of culture.
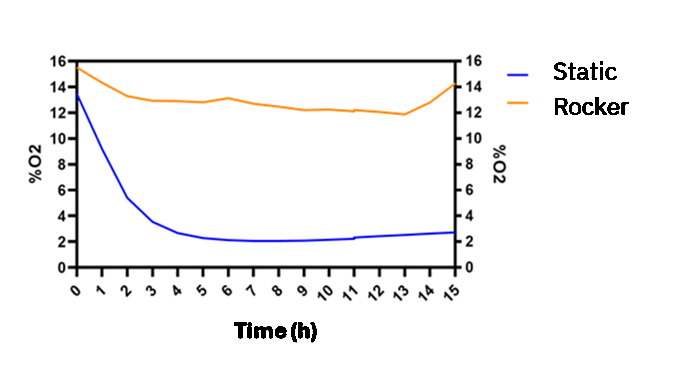
Figure 2. Graph of oxygen concentration evolution inside the Be-Flow microfluidic channel with a Caco-2 monolayer.
Morphology and layer integrity analysis
Moreover, cell monolayer morphology and integrity appeared significantly affected under static culture conditions, showing a better-preserved monolayer when oxygen and nutrient renewal are maintained with using a rocker. Figure 3.
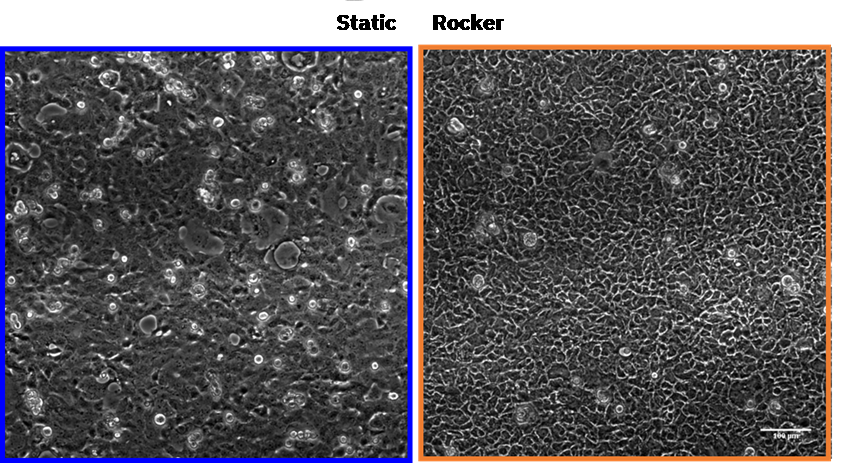
Figure 3. Brightfield optical micrographs of the Caco-2 cell line monolayer after 15 hours of culture under static and dynamic conditions. Scale bar 100 µm.
Overall, these results indicate that the use of gravity-driven flow is an important factor to consider in the early stages of organ-on-chip application before connecting to a flow control system. Precise control over channel dimensions (cell culture surface and cell number) and the renewal rate of the culture medium allow gas concentration regulation within Beonchip´s devices, generating gradients, ischemic processes, anaerobic environments, and more.
Bibliography
- Kim, D. & Herr, A. E. Protein immobilization techniques for microfluidic assays. Biomicrofluidics 7, 1–47 (2013).
- González-Lana, S. et al. Surface modifications of COP-based microfluidic devices for improved immobilisation of hydrogel proteins: long-term 3D culture with contractile cell types and ischaemia model. Lab Chip (2023) doi:10.1039/d3lc00075c.
- Keeley, T. P. & Mann, G. E. Defining physiological normoxia for improved translation of cell physiology to animal models and humans. Physiol. Rev. 99, 161–234 (2019).